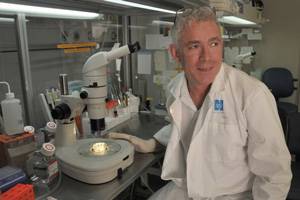
Once the focus of worldwide attention, genetic therapy’s dazzling potential has proven difficult to implement. However, a team at Hadassah is still researching healing through the manipulation of DNA—and they have had some surprising successes.
The idea of using genes to cure the incurable surfaced in the middle of the past century. By the 1990s, the first formal gene therapy studies were under way in the United States, and a few gene therapy drugs, like one for treating a viral disease of the eye in AIDS patients, had received Federal Drug Administration approval.
Overall, however, gene therapy seemed to fade from the radar. A rush of other medical technologies surged in, while at the same time, researchers learned just how great a distance there is between the concept of gene therapy and its actual clinical practice.
But gene therapy remains alive, well and under active investigation. To date, it has been used to treat over 10,000 people in more than 1,500 experimental studies worldwide. Researchers in 15 countries are exploring a swathe of gene therapy modalities to combat dozens of deadly diseases, from inherited disorders to cancers.
More than 90 of these gene therapy researchers are at the 10-year-old Goldyne Savad Institute of Gene Therapy at the Hadassah–Hebrew University Medical Center in Jerusalem. A relatively small and underfinanced group compared with those in the United States and Europe, its work is nonetheless landmark and has won the institute a respected name internationally.
“Our institute enjoys an important and unusual advantage,” says Dr. Eitan Galun, institute director and the medical center’s Sam and Ellie Fishman Chair in Gene Therapy. “We have all of the very diverse facilities necessary for gene therapy research concentrated in a single location.”
First, he explains, as part of a large medical center, the institute is enviably interdisciplinary. Its community of researchers includes experts in areas from stem cells to viral vectors, radiology to immunology, with cross-fertilization of ideas and techniques among them intense and ongoing.
Second, it has its own facility that makes the biological therapeutics it develops, and the facility follows GMP—good manufacturing practice—globally recognized production guidelines. And third, it has a clinical research ward, where products and techniques are brought to the bedside in phase 1 and 2 clinical trials immediately after they are approved. And all of this is in an academic environment, significantly supported by the entire medical center, from its administration to its imaging centers.
A lengthening string of patents bears testimony to the institute’s success. One of the more recent patents is for a molecule with potential to rescue patients in organ failure.
“Use of this molecule results from collaboration between us and a group headed by Stefan Rose-Jones at the University of Kiel in Germany,” says Dr. Galun. “They created it, and we discovered its ability to prevent injury and promote regeneration of injured tissues. This critical clinical application gives it potential to reverse what is now irreversible liver and kidney failure.”
The molecule is currently being tested in the salivary glands of animal models, where measuring its effect is relatively easy. “Nonfunctioning salivary glands create a distressing condition known as dry-mouth syndrome,” says Dr. Yitzchak Marmary, director of oral radiology at the Hebrew University–Hadassah School of Dental Medicine. “This is often an unwelcome side-effect of radiotherapy for cancers of the head, neck and oral cavity, in which the salivary glands are destroyed. Radiotherapists are in a Catch-22 here: The higher the radiation dose, the better the chance of cure—but the higher, too, the rate of dry-mouth syndrome and the lifelong suffering it bestows.”
The Hadassah team has injected the regenerative molecule into the salivary glands of animal models prior to radiation. They found that it protected the gland and prevented development of dry mouth.
“If this treatment works clinically, as we expect, we will not only be able to spare such patients severe and ongoing suffering, but also allow higher doses of radiation and so increase their chances of cure,” says Dr. Galun. “We’re now preparing for a clinical study and searching for the funding to support it.”
In their research into the molecule’s effect on the salivary gland, Hadassah investigators stumbled across something that, while having no impact on patient survival, contributes massively to their morale and well-being.
“While irradiated animals lose their hair just like human patients undergoing radiotherapy, some of the animal models with which we worked on dry-mouth syndrome kept their hair when irradiated,” says Dr. Jonathan Axelrod, a researcher at the institute. “We found that those that didn’t experience hair loss were failing to produce a certain protein.”
The researchers had discovered a protein that plays a central role in hair loss. They administered an antibody to the protein prior to radiotherapy in animal models that produce the protein. These animals kept their hair.
“We’re now preparing for clinical application of this antibody,” says Dr. Galun. “We expect it to prevent hair loss from chemo- as well as radiotherapy, and hope thus to psychologically ease cancer treatments.”
In most gene therapy protocols, delivering therapeutic genetic material to the site of the disease and having it function correctly once it gets there is the central stumbling block. A collaborative project between Hadassah and the Hebrew University of Jerusalem is developing a revolutionary delivery system that combines biotechnology with high technology.
“We’re working with an ultrafast laser that flashes on and off at a speed of a hundred millionth of a millionth of a second,” says Dr. Galun. “We’ve shown that such a laser effectively gets DNA into damaged cells. Once there, it interacts with the proper environment to make faulty cells express the correct proteins and doesn’t trigger uncontrolled cellular reactions.”
The technology was developed by a team headed by Aaron Lewis, who had established the Hadassah Laser Center and heads the Nanophotonics and Quantum Optics Research Laboratory at Hebrew University. “Clinical use of this laser is just beginning,” he says.
“One very important characteristic it has is that it can be focused beneath a surface with no side effects or collateral damage to overlying cells.” The main limitation of this low-power laser is that it cannot penetrate deeply into the body. The Hadassah-Hebrew University team has therefore used it in easily accessible skin and muscle tissue, and most recently in the eye—specifically, the region behind the retina or the lower-lying layers of the retina.
“There are 196 known genetic diseases that affect the retina,” says Dr. Yitzchak Hemo of Hadassah’s ophthalmology department, who, for the past six years, has been tracking culprit genes within the retina, a layer of tissue covering less than a square inch at the back of each eye. “The genes for 146 of these have been identified—among them, those for retinitis pigmentosa. In theory, in every retinal disease for which we know the genes, we can salvage the retina by introducing DNA.”
The DNA used to correct faulty retinal genes is genetically engineered in the Savad Institute’s GMP facility. It is naked DNA, a purified form of the molecule that is relatively cheap and easy to produce and, when taken up by malfunctioning retinal cells, repairs them. The DNA is delivered to the retinal cells through the iris by Lewis’s ultrafast laser methodology. “In animal models this has been very successful,” says Dr. Galun. “We’re now searching for funding to translate the technique into clinical studies.”
A completely different type of delivery system is at the center of another of the institute’s investigations. It is based on a finding made a decade ago by two Massachusetts researchers, Andrew Z. Fire and Craig C. Mello. Their discovery of RNA interference won them the Nobel Prize for Medicine in 2006.
RNA interference is a natural mechanism that controls the flow of genetic information. Messenger RNA (mRNA) carries the instructions our body sends for the manufacture of proteins from DNA. Fire and Mello found that when RNA molecules are generated in the cell in a certain form (as double-stranded pairs) they degrade mRNA that carries a matching genetic code. This RNA interference is probably there to help regulate gene expression, defend against viruses and control jumping genes (DNA sequences that move around in the genome). But RNA interference opens up exciting possibilities for gene technology, according to Dr. Galun.
“Theoretically,” he explains, “double-stranded RNA molecules, designed to target specific genes, can be introduced into the cell to silence genes that cause harm—those responsible, say, for high blood cholesterol levels, viral infections, cardiovascular diseases, cancer, endocrine disorders and more.”
At Hadassah, researchers are exploring the use of this technology against cancers. “We know how certain cancers develop,” says Dr. Galun. “Pancreatic cancer is an example. Over 90 percent of pancreatic cancer patients have a specific mutation. If we can target these mutated genes with specific double-stranded RNA, we’ll significantly slow growth of the tumor.”
Here, as in so much of gene therapy, the main problem is delivery. How do you get the killer RNA to the cancerous genes? “We believe we’ve found a way,” says Dr. Galun. “In collaboration with Dr. Amotz Shemi [director of Silenseed Ltd], we have created a small local device that is implanted into the patient, where it sheds RNA over weeks and months. A start-up company within Hadassah, Silenseed, is developing the device, initially for patients with pancreatic cancers, with plans to address cancer of the prostate and glioblastoma [a form of brain tumor] at a later stage. We’ve shown that it’s effective in tissue culture and in animal models and…we are hoping to begin clinical trials at Hadassah next spring.”
A defect in any one of the 35,000 to 40,000 genes that each of us carries can lead to disease. Each day, we are getting closer to a time when healthy replacements for impaired or missing genes will be routinely inserted; a time when doctors, instead of struggling to keep up with the symptoms, will anticipate the root cause of illness and have the tools to treat it.